One of the most promising business cases for cloud and Internet of Things (IoT) services is environmental monitoring to help ensure a stable indoor growing environment for the localized cultivation of non-native crops such as fruits, nuts, vegetables and herbs.
Moving Horticulture and Indoor Cultivation to the Cloud
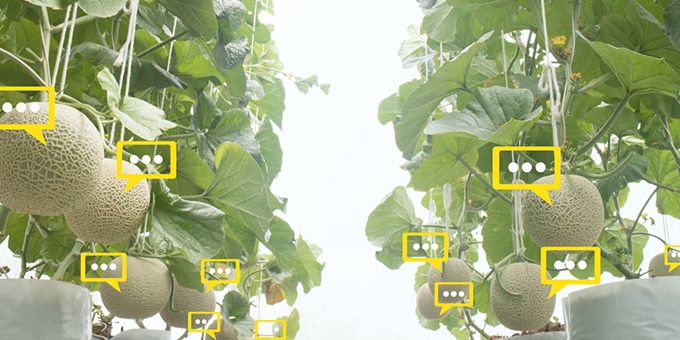
Aloke Barua, Sr. Product Marketing Engineering | Microchip Technology Inc.
One of the most promising business cases for cloud and Internet of Things (IoT) services is environmental monitoring to help ensure a stable indoor growing environment for the localized cultivation of non-native crops such as fruits, nuts, vegetables and herbs. Cloud-connected IoT networks will enable the use of efficient pre- and post-harvest management systems for horticulture and indoor cultivation applications and fuel the birth and growth of a large-scale Controlled Environmental Agriculture (CEA) industry that is ethical, sustainable and ultimately profitable. These systems will leverage IoT sensors that continually measure and report environmental data, as well as trigger actions or commands when adjustments are required. Growers will need to understand best practices for specifying and deploying these systems, including the critical requirement that they implement encryption and authentication technologies so that hackers cannot take control to harm operations or steal valuable data.
The Case for Localized, Indoor Cultivation
Before exploring how to develop cloud-connected localized cultivation systems, it’s important to understand the potential benefits of indoor cultivation. The three primary benefits include:
More Produce Availability
Global demand for fruits, nuts, vegetables and herbs that are non-native to a specific geography can be addressed more rapidly and efficiently if these crops are grown locally in that geography. It will also help reduce food miles – while this may not make operations less carbon-intensive, it will certainly help increase food security and the availability of produce that would otherwise be transported long distances from somewhere else. Other side benefits include an extended growing season that makes it possible for a given food crop to be available 12 months of the year, and the ability to maintain efficient global food production despite the Earth’s erratic climatic conditions.
Reduced Habitat Destruction
An increase in CEA or indoor cultivation of non-grain crops could also reduce habitat destruction. While this may be controversial, there are sound reasons why agriculture is often overlooked as a threat to habitats and the environment, with most attention focused on land development and deforestation by the logging industry. According to the World Wide Fund for Nature (WWF), “the Earth loses 18.7 million acres of forests per year” based on these three major contributors and “around 50 percent of the world’s habitable land has been converted to farming land.” Major land uses include grazing and housing livestock, massive farming operations and growing vegetables and grains for human consumption, with more than a third dedicated to the production of livestock feed crops such as corn, barley, oats, sorghum and soya. It is becoming clear that farming techniques that consume vast amounts of land are unsustainable.
Healthier Diets
In addition to reducing how much land is required for traditional farming techniques, CEA and indoor cultivation can help foster healthier diets. It is a well-established medical fact that while humans are omnivorous, they fare better with a diet that is biased toward a mixture of plants, fruits and nuts with reduced meat consumption. Broader adoption of this diet will lead to a significant reduction in the production of livestock feed, and thus enable a fundamental shift toward the creation of a sustainable lifestyle for the global population. Additionally, this could reduce the quantity of livestock being reared, which could reduce food transportation requirements and reduce greenhouse gas (GHG) emissions. According to the Food and Agriculture Organization (FAO) of the United Nations, global livestock produces GHG emissions that represent 14.5 percent of all anthropogenic, or human-induced, GHG emissions.
Large-scale CEA and indoor cultivation are in their infancy. While the more common approach is to deploy indoor structures such as greenhouses across wide tracts of land, there is an increasing trend toward the construction of bespoke vertical farming structures, including re-purposed factories or warehouses containing several floors. This enables a more efficient and pragmatic use of real estate and land for the growth of non-grain crops. Vertical farming is also well-suited for raising poultry as a potentially more viable source of meat.
There are several prerequisites for implementing large-scale CEA and indoor cultivation. Reproducing and maintaining native conditions requires the grower to regularly monitor parameters such as heat, artificial lighting, humidity, soil moisture and, in the case of hydroponics/aeroponics, the water nutrients. Serious producers will have many buildings housing enclosed environments, each of which must be optimized for these growth conditions. This requires the use of a cloud-connected sensor, or sensors for continually measuring the environmental condition levels over time and reporting data to a central monitoring station.
Best Practices for System Deployment
The first step is to create a log of the native or non-native outdoor conditions that will be used as a benchmark to make the necessary environmental adjustments as required.
Next, a decision must be made about the type of network to deploy. With the growing availability of the IoT for connecting sensors, it makes sense to deploy a network governed by a central hub or gateway that communicates with a local controller or computer (see Figure 1). The controller is used to upload data to the cloud for further analytics. The cloud can be proprietary or offered as a service by either an established or emerging provider.
Figure 1: Typical implementation of an IoT ecosystem
A grower may decide that it is not necessary to react instantaneously to sensor data. In these cases, the cloud can issue commands or actions in the timeframe deemed acceptable. However, if minimal or zero latency is required between when sensor data is sent, and the central computer issues an action or command, then the grower/producer can take the intermediate step of using an edge controller between the gateway and the cloud to speed the time from analytics to action. Ultimately, the more accurately the environment is controlled, the better the crop grows.
The edge computing implementation can be split into back- and front-end sections, each playing an important role in optimizing the closed environment’s crop production. The back-end section contains the edge computing and cloud computing elements while the front-end section contains the sensor network and gateway elements. An ecosystem of hardware suppliers and system integrators will emerge to deliver all the elements required to support this solution architecture for a growing number of IoT implementations and use cases across various industries. One particularly important element is the sensor network as this will be positioned closest to the crop and is responsible for monitoring its environment and harvesting the data for transmission to the gateway. It is also crucial that each of many individual sensor nodes is simple, reliable, easy to service, operates with very low power to extend battery life, and can communicate with the gateway and ultimately the cloud service provider using various wireless connectivity methods.
The preferred wireless connectivity element will be either Bluetooth® Low Energy (BLE) or the new 802.11ah low-power Wi-Fi® standard. This will ensure that solutions can operate over unlicensed bands and communicate across the typical 10- to 100-meter (m) distances of indoor cultivation. The 802.11ah standard has the longest reach, up to 1 kilometer (km). Data rates for BLE and 802.11ah Wi-Fi are 10 kilobits per second (Kbps) to 10 Mbps and 50 Kbps – 100 Kbps, respectively, providing ample bandwidth for the various parameter data that is being measured.
It is essential that the sensor node also have robust security. As in any business, there will be many farmers or large conglomerates getting involved in the indoor cultivation or CEA industry on a large scale. Any information that can help one supplier gain a competitive edge over another means higher revenue and profits. The desire and ability to hack into a wireless network to acquire data that delivers this advantage is widely accepted, recognized and understood. The best way to mitigate the risk is with a hardware-based solution that can encrypt as well as authenticate both the data and the node. Firmware or software approaches are widely acknowledged to be vulnerable to hackers.
Editors Recommendation "Universal Automation in Agriculture"
Designers and manufacturers of sensor nodes will base their offerings on silicon solutions that help make the finished system design easy to use, modular and updatable, with low-power operation, robust security and the flexibility to support required wireless connectivity options. These suppliers also actively collaborate with prominent cloud service providers. If the solution must be cloud-agnostic, the supplier should be capable of configuring the solution to communicate with a propriety cloud with all the required capabilities.
One example of this type of solution is the Google Cloud IoT Core development platforms offered by Microchip Technology (see Figures 2 and 3). The development boards combine a microcontroller, secure element and fully certified Wi-Fi network controller to offer the most simple and effective way to connect sensor nodes to Google’s Cloud IoT Core platform. Users can make a direct connection to Google Cloud, pre-provisioned with a free sandbox account, or virtual testing environment, to see light and temperature data. Additional sensors can be optionally attached through the use of widely available add-on Click™ boards from MikroElektronika that make it easy to add capabilities to a design.
Figure 2: The AVR-IoT WG Development Board features a powerful AVR® microcontroller (MCU), secure element and Wi-Fi network controller.
Figure 3: The PIC-IoT WG development board features an eXtreme Low-Power (XLP) PIC® MCU, secure element and Wi-Fi network controller.
A Better Way to Feed the Planet
CEA and indoor cultivation offer the promise of more secure, reliable and efficient global food production in a significantly smaller geographic footprint than is possible with traditional farming methods. Realizing this promise requires environmental monitoring technology that, on a large scale, can ensure a stable indoor growing environment for non-native crops. Among the most important of these technologies is the sensor network that harvests and transmits environmental monitoring sensor data to and from the cloud for processing and analytics. Rapid development solutions have been introduced with leading cloud providers to meet these requirements, providing simple and flexible ways to support a variety of CEA and indoor cultivation applications and use cases.
Glossary of Terms
Agriculture: The science or practice of farming, including cultivation of the soil for the growing of crops and the rearing of animals to provide food, wool and other products. Primarily done on large areas of land.
Horticulture: The science or practice of growing fruits, herbs, vegetables, nuts, ornamental plants and flowers. It is generally classified as a subdivision of agriculture and has the same timeline for its history and development. Unlike agriculture, horticulture is practiced on smaller, enclosed areas and usually involves a wide variety of crops whereas agriculture focuses on one main crop. Its financial viability on a large scale may require that it be focused on a single crop per enclosed structure, to minimize the difference in growth environments required per crop thereby reducing the complexity of the support infrastructure.
Controlled Environment Agriculture (CEA): A technology-based approach toward food production. The aim of CEA is to protect and maintain optimal growing conditions for a crop throughout its development. Production takes place within an enclosed growing structure such as a greenhouse or building. Plants are often grown using hydroponic methods in order to supply the proper amounts of water and nutrients to the root zone. CEA optimizes the use of resources such as water, energy, space, capital and labour. CEA technologies include hydroponics, aquaculture, and aquaponics. Controllable variables include: Temperature (air, nutrient solution, root-zone); humidity (%RH); carbon dioxide (CO2); light (intensity, spectrum, interval); nutrient concentration (parts per million, or PPM, and electrical conductivity, or EC); fertilizers; and nutrient pH (acidity). CEA facilities can range from fully automated greenhouses with computer controls for watering, lighting and ventilation, to low-tech solutions such as plastic film or coverings called cloches that are placed on field-grown crops, and plastic-covered tunnels.
Vertical Farming: The practice of producing food in vertically stacked layers, such as in a skyscraper, used warehouse, or shipping container, and controlling environmental factors using indoor farming techniques and CEA technology. Vertical farming techniques are often similar to greenhouses, augmenting natural sunlight with artificial lighting and metal reflectors.
The Cloud: A metaphor for the internet, the cloud stores information/data on physical or virtual servers that are maintained and controlled by a cloud computing provider such as Amazon with its AWS product, Microsoft® with Azure and Google with its Google Cloud Platform™ (GCP) service.
Cloud Computing: The sharing of resources, software and information through a public, private or hybrid network so that companies can cut down on overhead costs by conducting the computer-related aspects of their business virtually. Appropriate for big data analytics and useful in applications where reaction time is not critical.
Edge Computing: The process of performing data processing at the edge of the network, near the source of the data, to reduce roundtrips to the cloud and associated latency or communications bandwidth. Edge computing is often used in industrial IoT (IIoT) applications that require instantaneous decision-making. Edge and cloud computing are complementary and, depending on the use case, can be used in conjunction with each other.
IoT: The Internet of Things, at its very basic level, refers to the connection of everyday objects to the internet and to one another, including sensors and actuators connected by networks to computing systems. By combining these connected devices with automated systems, it is possible to harvest and analyse this information and invoke actions from it.
If you like this article you may like "The Age of Autonomy: Robotic Solutions for the Horticulture Industry"
The content & opinions in this article are the author’s and do not necessarily represent the views of AgriTechTomorrow
Comments (0)
This post does not have any comments. Be the first to leave a comment below.
Featured Product
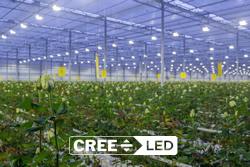